by Jack Hoeniges
If you’re a bit of an optimist, you probably believe that at some point in the future, far or near, we’ll live in a world that is carbon neutral. To make this future a reality, there’s one type of energy among the top technologies that is expected to get us there: solar. Indeed, residential and utility scale solar photovoltaic (PV) installations are more prevalent now than ever. Since 1980, the cost per watt of electricity generated via solar PV has dropped from $70 to a mere 36 cents, and it’s still falling; in the last decade alone, cost has decreased by 70% [1,2]. In 2019, solar accounted for 40% of the generating capacity added to the United States’ energy grid [2].
But solar technology had a long journey to get where it is now. The phenomenon that allows solar panels to exist was first observed by Heinrich Hertz in 1887, who noted that shining a light on two electrodes could intensify the spark generated between them [3]. From there, it took almost another twenty years and the most renowned scientist of the 20th century – Albert Einstein – to explain the phenomena observed by Hertz, now known as the photoelectric effect; an achievement for which he would win the Nobel Prize. Einstein published his paper in 1905, with it, he gave us a little seedling of a technology that would blossom over the course of a hundred years into the solar panels we know and use today.
But as it turns out, the natural world had already beat us to the punch. Plants have been using photosynthesis to harvest and store energy since before humans existed. When we eventually got around to making use of this virtually infinite energy source, scientists and engineers contrived a seemingly simple black rectangle, perched unassumingly on your neighbors’ roof. It is essentially a cocktail more elaborate than one you’d find at a hipper-than-thou speakeasy: two parts rare earth metals, one part Einstein, a generous dash of quantum mechanics, aged for nearly a century and a half, all just so you can stream The Office for the umpteenth time. We went through quite the trouble just to capture solar energy that was already being captured all around us. Therein lies the beauty of biofuels. While photovoltaics will undoubtedly play a crucial role in our carbon neutral future, biofuels take advantage of nature’s solar panel and our existing infrastructure to create a promising avenue to carbon neutrality.
Despite the natural elegance of photosynthesis, biofuels are tricky. Large scale implementation of biofuels comes with a unique set of challenges that we are already facing. One example is the less-than-elegant way biofuels are currently integrated into our energy economy. Currently an Environment Protection Agency (EPA) mandate stipulates that fuel blends must contain a certain percentage of biofuels, creating reliable demand for corn to produce ethanol. But is demand created solely by an EPA mandate a sustainable model for farmers? Add to this the consumer confusion around ethanol-containing blends such as E10 (i.e. “why is this ‘green’ fuel causing my car to get worse gas mileage?”) and we’re off to a bad start.
Then there are questions of air quality. Will big cities and disadvantaged communities be any better off if our hydrocarbons come from the plants rather than the ground? Will clean combustion technologies advance – or are they already advanced enough – to allow us to completely alleviate the concerns that come along with powering so much of the transportation sector by combustion, a technology that’s now several centuries old? And even if high yield, fast-growing bio-feedstocks like microalgae are used, how much fertilizer would be needed in order to create a meaningful impact on the energy sector? Producing enough fertilizer to meet such a demand will be no easy (or for that matter, low emissions) task. Making biofuels feasible is not just a task of bioprocess, genetic, and chemical engineering, it’s also a social, political, and economic challenge. Failing to address even one piece of the puzzle puts the final picture at risk.
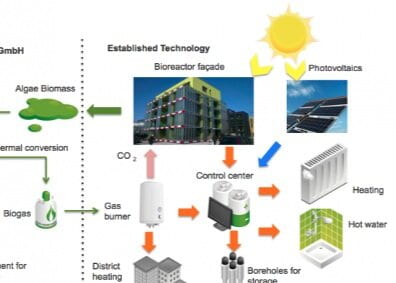
Schematic of the bio-energy loop employed by the BIQ Building [4]
Currently, a futuristic glimpse of how biofuels might fit into the energy economy of the future while addressing all these pillars of sustainability is already available In Germany. The Bio-Intelligent Quotient Building (BIQ) in Hamburg is a building that has integrated microalgae production – a third generation biofuel feedstock – into its very architecture. The bio-façade is made of vertical flat plate photobioreactors, giving the building the look of an enormous, futuristic green lava lamp. But beyond just looking cool, these bio-façades shade the building, which can help reduce energy consumption, and they contain microalgae cultures that take in sunlight and carbon dioxide to produce lipids for biogas. The biogas is then used for district heating and the CO2 produced during combustion is looped back to the microalgae to produce more of the bio-feedstock [4].
Compared to conventional biomass, this type of biofuel production has several advantages: there’s no competition for land or food usage (as is the case with corn and ethanol), the carbon sink (microalgae) is integrated with the corresponding carbon source (biogas burner) ensuring the CO2 produced is reabsorbed immediately, and the opportunities for innovation in a set up like this are plentiful. In a city like LA for example, bio-façades could be used as a means of reducing pollution levels locally, and the resulting fuel could be sold to the hordes of semis that take goods from the Port of LA to the rest of the US every day. In a carbon neutral future, high rates of atmospheric CO2 near cities could be mitigated with urban bio-façades, thus turning the air pollution in urban areas into fuel to power the rest of the country.
Biofuels are by no means a catch-all; few believe that there will be a single silver-bullet solution to weaning our global economy off our carbon dependence. Increasingly affordable battery electric vehicles will likely cause personal transportation to transition to electric power, but sectors that are more difficult to electrify, such as freight and aviation, may need biofuels to reach carbon-neutrality.
Much of the awakening of the 20th century was realizing how far reaching the impact of new technologies can be; our responsibility and that of future generations will be to take this lesson to heart and confront the climate crisis by seeing current and developing technologies more holistically. With a shortsighted focus on quick fixes, biofuels will be destined for failure, but with an approach that is honest about the strengths and weaknesses of biofuels and conscientious of the social, economic, and environmental impacts, they represent a promising, flexible, and elegant weapon in our arsenal in the battle with climate change.
Sources
- Gloystein, Henning. “Large-Scale Solar Power Set for Double-Digit Growth: Goldman Sachs.” Reuters, Thomson Reuters, 14 Mar. 2019, www.reuters.com/article/us-solar-power-goldman-sachs/large-scale-solar-power-set-for-double-digit-growth-goldman-sachs-idUSKCN1QV0GI.
- “Solar Industry Research Data.” SEIA, www.seia.org/solar-industry-research-data.
- Mari, Lorenzo. “A History of the Photoelectric Effect and Its Role in Solar PV – Technical Articles.” EEPower, 8 May 2020, eepower.com/technical-articles/a-history-of-the-photoelectric-effect-and-its-role-in-solar-pv/.
- Fytrou-Moschopoulou, Arla. “The BIQ House: First Algae-Powered Building in the World.” Build Up, 29 Apr. 2015, www.buildup.eu/en/practices/cases/biq-house-first-algae-powered-building-world.
Jack is part of the 2018-2019 INFEWS program cohort and a PhD candidate in the Department of Mechanical and Aerospace Engineering at UCLA. His research focuses on water treatment and production of food and biofuel via microalgae
This is a pop science article and is part of the INFEWS Social Media Series.